Scientists have found the first evidence of a source of high-energy cosmic neutrinos, ghostly subatomic particles that can travel unhindered for billions of light years from the most extreme environments in the universe to Earth.
“For years, we’ve had a long list of potential sources for high-energy neutrinos. Now we have a specific source—blazars—that we can look at very carefully.”
The observations, from the IceCube Neutrino Observatory at the Amundsen–Scott South Pole Station in coordination with telescopes around the globe and in Earth’s orbit, help resolve a more than a century-old riddle about what sends subatomic particles such as neutrinos and cosmic rays speeding through the universe.
Since their first detection over one hundred years ago, cosmic rays—highly energetic particles that continuously rain down on Earth from space—have posed an enduring mystery: What creates and launches these particles across such vast distances? Where do they come from?
Because cosmic rays are charged particles, their paths cannot be traced directly back to their sources due to the magnetic fields that fill space and warp their trajectories. But the powerful cosmic accelerators that produce them will also produce neutrinos. Neutrinos are uncharged particles, unaffected by even the most powerful magnetic field. Because they rarely interact with matter and have almost no mass—hence their nickname “ghost particle”—neutrinos travel nearly undisturbed from their accelerators, giving scientists an almost direct pointer to their source.
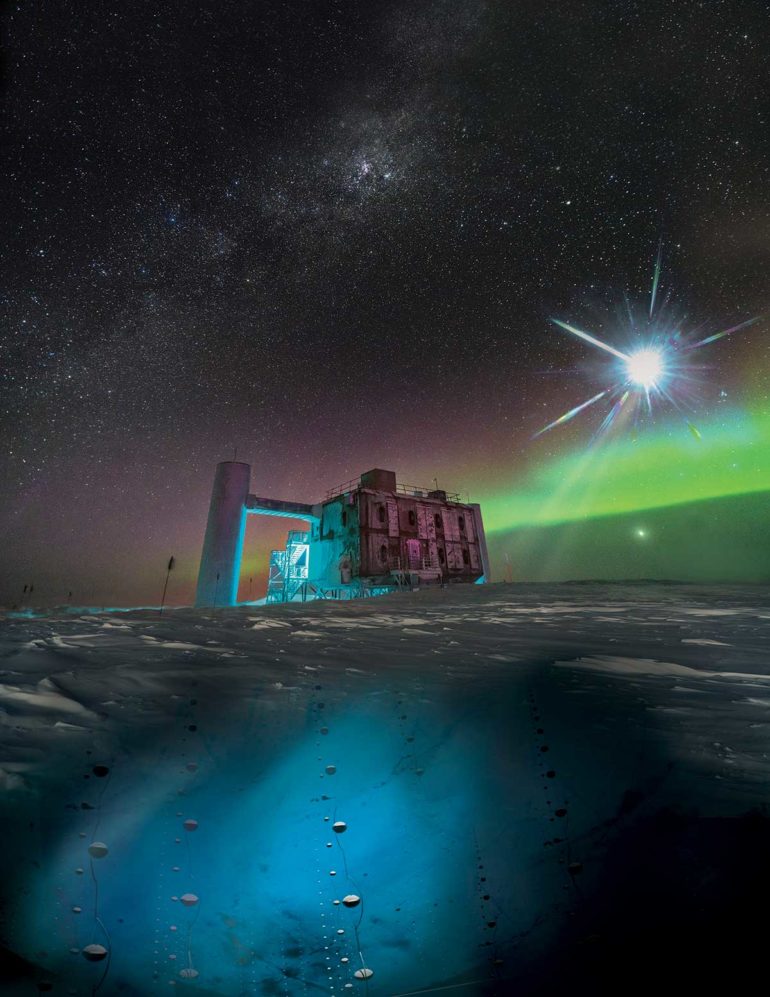
Two new papers (first, second) in the journal Science for the first time provide evidence for a known blazar as a source of high-energy neutrinos detected by the IceCube observatory. This blazar, designated by astronomers as TXS 0506+056, was first singled out following a neutrino alert sent by IceCube on September 22, 2017.
“IceCube-170922A—a high-energy neutrino detected by IceCube on September 22, 2017—had an energy of 300 trillion electron volts and a trajectory pointing back to a small patch of sky in the constellation Orion,” says coauthor Azadeh Keivani, a postdoctoral scholar at Penn State.
“The era of multi-messenger astrophysics is here. Each messenger gives us a more complete understanding of the universe and important new insights into the most powerful objects and events in the sky,” says NSF director France Córdova. “Such breakthroughs are only possible through a long-term commitment to fundamental research and investment in superb research facilities.”
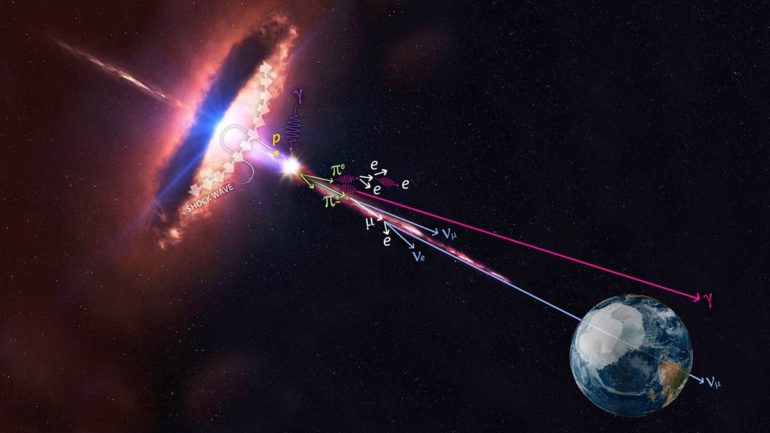
A blazar is a galaxy with a super-massive black hole at its core. A signature feature of blazars is twin jets of light and elementary particles emitted from the poles along the axis of the black hole’s rotation. In this blazar, one of the jets points toward Earth. This blazar is situated in the night sky just off the left shoulder of the constellation Orion and is about four billion light years from Earth.
“Scientifically, this is very good news,” says Ignacio Taboada, an associate professor in Georgia Tech’s School of Physics and member of the Center for Relativistic Astrophysics also at Georgia Tech. As leader of the “Transients Science Working Group” within IceCube, he oversaw all the studies that inquired on the correlation TXS 0506+056’s gamma ray flare and the neutrino alert of September 22, 2017. “For years, we’ve had a long list of potential sources for high-energy neutrinos. Now we have a specific source—blazars—that we can look at very carefully.”
Georgia Tech PhD student Chun Fai (Chris) Tung contributed to the publications by reconstructing archival IceCube data searching for very-high energy neutrinos that might be correlated with blazars other than TXS 0506+056.
“At the highest energies, the universe is essentially opaque to very high energy gamma rays, and the farther away you are, the more opaque the universe is,” Taboada says. “If the blazar had been closer we likely would have seen it with HAWC,” the Higher Altitude Water Cherenkov gamma-ray observatory in central Mexico.
One in a million
Equipped with a nearly real-time alert system—triggered when a very high-energy neutrino collides with an atomic nucleus in the Antarctic ice in or near the IceCube detector—the observatory broadcast coordinates of the September 22 neutrino alert to telescopes worldwide for follow-up observations.
Two gamma-ray observatories, NASA’s orbiting Fermi Gamma-ray Space Telescope and the Major Atmospheric Gamma Imaging Cherenkov Telescope, or MAGIC, in the Canary Islands, detected a flare of high-energy gamma rays associated with TXS 0506+056, a convergence of observations that convincingly implicated the blazar as the most likely source.
Fermi was the first telescope to identify enhanced gamma-ray activity from TXS 0506+056 within 0.06 degrees of the IceCube neutrino direction. In a decade of Fermi observations of this source, this was the strongest flare in gamma rays. A later follow-up by MAGIC detected gamma rays of even higher energies.
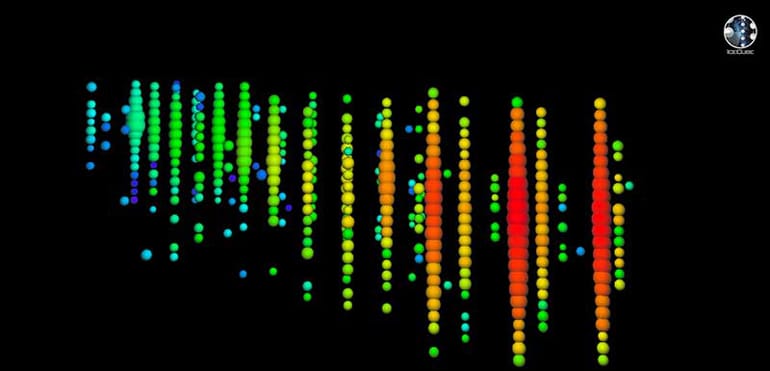
These observations prove that TXS 056+056 is one of the most luminous sources in the known universe and, thus, add support to a multimessenger observation of a cosmic engine powerful enough to accelerate high-energy cosmic rays and produce the associated neutrinos. Because neutrinos interact so weakly with matter, IceCube detected only one out of many millions that sailed through Antarctica’s ice on September 22.
Bolstering these observations are coincident measurements from other instruments, including optical, radio, and X-ray telescopes. “The ability to globally marshal telescopes to make a discovery using a variety of wavelengths in cooperation with a neutrino detector like IceCube marks a milestone in what scientists call multi-messenger astronomy,” says Halzen.
A mystery since 1912
Austrian physicist Victor Hess showed, in 1912, that ionizing particles detected in the atmosphere arrive from space. These cosmic rays are the highest energy particles ever observed, with energies up to a hundred million times the energies of particles in the Large Hadron Collider at CERN in Switzerland, the most powerful human-made particle accelerator.
These extremely high-energy cosmic rays can only be created outside our galaxy and their sources have remained a mystery until now. Scientists had speculated that the most violent objects in the cosmos, like the mysterious gamma ray bursts, colliding galaxies, and the energetic black hole cores of galaxies known as active galactic nuclei, such as blazars, could be the sources.
“Fermi has been monitoring some 2,000 blazars for a decade, which is how we were able to identify this blazar as the neutrino source,” says Regina Caputo, the analysis coordinator for the Fermi Large Area Telescope collaboration. “High-energy gamma rays can be produced either by accelerated electrons or protons. The observation of a neutrino, which is a hallmark of proton interactions, is the first definitive evidence of proton acceleration by black holes.”
“Now, we have identified at least one source of cosmic rays because it produces cosmic neutrinos. Neutrinos are the decay products of pions. In order to produce them, you need a proton accelerator,” says Halzen.
Neutrino experiments could rewrite Standard Model of Physics
Cosmic rays are mostly protons and are sent speeding across the universe because the places where they are created act in the same way as particle accelerators on Earth, only they are far more powerful. “Theories predict that the emission of neutrinos will be accompanied by the release of gamma rays,” explains Razmik Mirzoyan, the spokesperson of the MAGIC Collaboration. But there are still a lot of questions on how blazars could accelerate particles to the highest energies. “Gamma rays provide information on how the ‘power plants’ in supermassive black holes work,” adds Mirzoyan.
Neutrinos ‘hardly ever stop to interact’
As the latest astrophysical messenger to enter the game, neutrinos bring crucial new information to uncovering the inner workings of these cosmic ray accelerators. In particular, measurements of neutrinos can reveal the mechanisms for particle acceleration of the proton beam in the densest environments that even high-energy gamma rays may not escape.
“For the most part, neutrinos go through everything and hardly ever stop to interact.”
Following the September 22 detection, the IceCube team quickly scoured the detector’s archival data and discovered a flare of over a dozen astrophysical neutrinos detected in late 2014 and early 2015, coincident with the same blazar, TXS 0506+056. This independent observation greatly strengthens the initial detection of a single high-energy neutrino and adds to a growing body of data that indicates TXS 0506+056 is the first known accelerator of the highest energy neutrinos and cosmic rays.
Detecting high-entry astrophysical neutrinos—particles from outside our galaxy—is no easy task. These particles pass through the Earth as if it were glass and are only detectable when they interact with atomic protons and neutrons that are massive enough to stop them. “For the most part, neutrinos go through everything and hardly ever stop to interact,” says Taboada.
A team at the University of Wisconsin-Madison operates the IceCube Neutrino Observatory, which the National Science Foundation primarily funds.
About 20 observatories on Earth and in space have participated in the identification of what scientists deem to be a source of very high-energy neutrinos and, thus, of cosmic rays. Several follow-up observations are detailed in a few other papers that are also being published.
Source: Georgia Tech (adapted from the original release via IceCube)