The signal from a fast radio burst—an enigmatic blast of cosmic radio waves lasting less than a millisecond—is helping researchers characterize the diffuse gas in the halo of a massive galaxy.
Their findings reveal that the massive galaxy sports an unexpectedly inactive halo, with a very low density and weak magnetic field.
This discovery gave scientists a rare glimpse of galactic halos, which contain clues to how gas feeds into and is expelled from galaxies. Astronomers think these galactic halos could be more massive than the galaxies themselves, and that studying them could reveal how galaxies form.
Glimpsing galactic halos
“The signal from this fast radio burst went just by the edge of a galaxy, giving us our first glimpse into the structure of the most diffuse halo gas that surrounds galaxies,” says coauthor Matthew McQuinn, an assistant professor of astronomy at the University of Washington. “This event demonstrates a technique that is going to transform our understanding of this diffuse gas.”
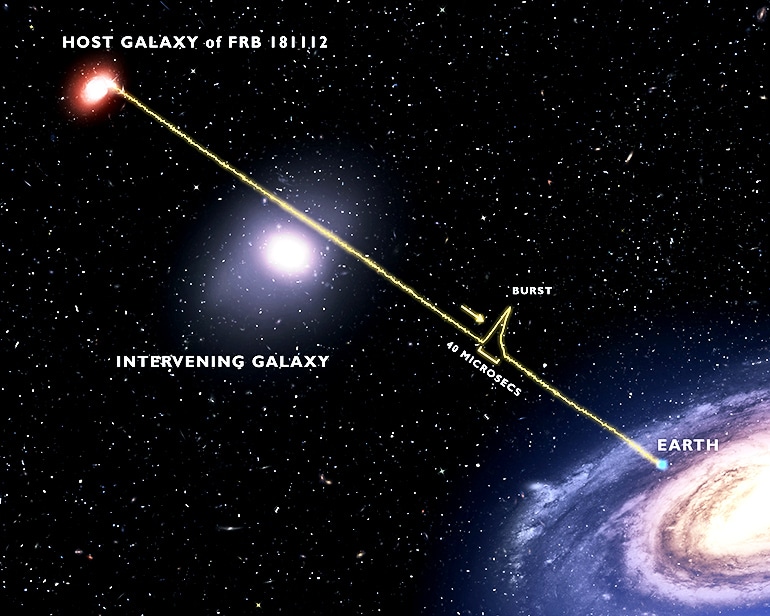
McQuinn proposed this technique in a 2014 paper in the Astrophysical Journal Letters.
Vast halos of low-density gas extend far beyond the luminous parts of galaxies where the stars are concentrated. Although this hot, diffuse gas makes up more of a galaxy’s mass than stars, and may make up as much as half of the gas in the universe, it is nearly impossible to see. In November 2018, astronomers detected a fast radio burst that passed through the halo of a massive galaxy on its way toward Earth, allowing them to learn about the nature of the halo gas from this elusive radio signal.
Astronomers still don’t know what produces fast radio bursts, and could only recently trace some of these very short, very bright radio signals back to the galaxies in which they originated. Researchers detected and localized the November 2018 burst, named FRB 181112, with the instrument that pioneered this technique, the Australian Square Kilometre Array Pathfinder, a radio telescope in Western Australia that the Commonwealth Scientific and Industrial Research Organisation operates. Follow-up observations with European Southern Observatory’s Very Large Telescope in Chile identified not only its host galaxy but also a bright galaxy in front of it.
“When we overlaid the radio and optical images, we could see straight away that the fast radio burst pierced the halo of this coincident foreground galaxy and, for the first time, we had a direct way of investigating this otherwise invisible matter surrounding this galaxy,” says coauthor Cherie Day at Swinburne University of Technology.
A fossil record in space
A galactic halo contains both dark matter and baryonic matter—or ordinary matter—which is expected to be mostly hot ionized gas. While the luminous part of a massive galaxy might be around 30,000 light-years across, its roughly spherical halo is ten times larger.
Halo gas fuels star formation as it falls in toward the center of the galaxy, while other processes, such as supernova explosions, can eject material out of the star-forming regions and into the galactic halo. One reason astronomers want to study the halo gas is to better understand these ejection processes, which can shut down star formation.
“The halo gas is a fossil record of these ejection processes, so our observations can inform theories about how matter is ejected and how magnetic fields are threaded through galaxies,” says lead author J. Xavier Prochaska, a professor of astronomy and astrophysics at the University of California, Santa Cruz.
Contrary to expectations, the results of the new study indicate a very low density and a relatively feeble magnetic field in the halo of this intervening galaxy.
“The radio signal was largely unperturbed by the galaxy, which is in stark contrast to what previous models predict would have happened to the burst,” says Prochaska.
Super quick pulses
FRB 181112 consisted of several pulses, each lasting less than 40 microseconds—ten thousand times shorter than the blink of an eye. McQuinn helped lead the interpretation of this signal. The short duration of the pulses puts an upper limit on the density of the halo gas, because passage through a denser medium would lengthen the radio signals.
The researchers calculated that the density of the halo gas must be less than a tenth of an atom per cubic centimeter, which is equivalent to several hundred atoms in a volume the size of a balloon.
“Like the shimmering air on a hot summer’s day, the tenuous atmosphere in this massive galaxy should warp the signal of the fast radio burst,” says coauthor Jean-Pierre Macquart, an astronomer at the International Center for Radio Astronomy Research and associate professor at Curtin University. “Instead we received a pulse so pristine and sharp that there is no signature of this gas at all.”
The density constraints also limit the possibility of turbulence or clouds of relatively cool gas within the halo, which astronomers have theorized might be present in halos.
The FRB signal also yields information about the magnetic field in the halo, which affects the polarization of the radio waves. Analyzing the polarization as a function of frequency gives a “rotation measure” for the halo, which the researchers found to be about a billion times weaker than an ordinary refrigerator magnet, says Prochaska.
Since these results come from only one galactic halo, the team cannot say whether the low density and magnetic field strength are unusual or if previous studies of galactic halos have overestimated these properties. But radio telescopes can use fast radio bursts to study many more galactic halos and resolve their properties.
The study appears in Science.
Additional coauthors are from UC Santa Cruz; the Swinburne University of Technology; Macquarie University; the Commonwealth Science and Industrial Research Organisation; the North Carolina State University; the Gwangju Institute of Science and Technology; the University of Sydney; and the Pontificia Universidad Católica de Valparaíso.
Funding for the work came from the National Science Foundation, the Australian Research Council, and the Pontificia Universidad Católica de Valparaíso.
Source: University of Washington