Researchers have developed a technique that allows them to tag specific parasite genes with fluorescent colors to better track them throughout the parasite’s complicated life cycle.
“It opens up a whole range of new experiments…”
Microbiologists will now be able to more easily identify malaria’s weak spots, providing drug developers with new targets for anti-malaria drugs and stay ahead of the parasite’s frightening ability to build resistance.
Crucially, it will also make it easier for researchers to develop potential vaccines.
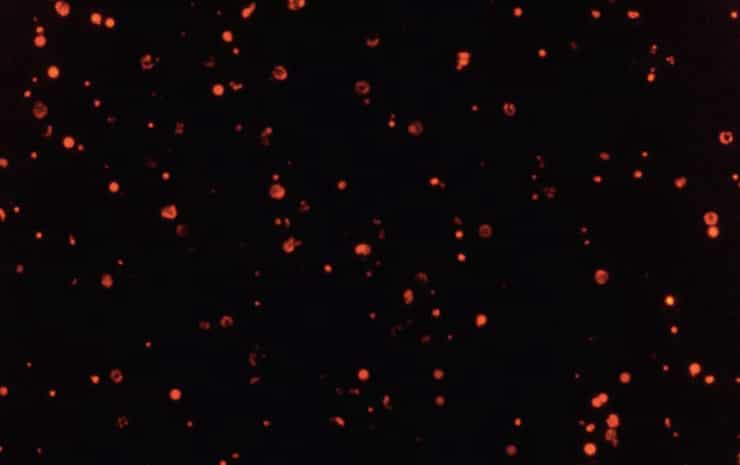
“It opens up a whole range of new experiments researchers can undertake, targeting different genes and processes to try to better understand what is actually happening at different stages,” says Dean Goodman, of the University of Melbourne’s School of BioSciences.
Up until now it has been extremely difficult to track the role of different malaria genes because the parasite radically changes form to adapt to surviving first in mosquitoes and then in mammalian hosts like us.
Scientists around the world particularly want to know how malaria adapts when it reaches the liver, which is the critical stage where it learns how to infect the blood stream and cause symptoms. But now researchers will be able to simply track the fluorescent parasites in order to work out when a specific gene is critical or not during the parasite’s life cycle.
“Once we understand the critical process at work, we will be in a much stronger position to produce drugs that can interfere with these processes and block the parasite from adapting, effectively killing it in the liver before it can get into the blood.”
The critical gene
The technique has successfully shown that the malaria’s ferrochelatase (FC) gene is critical for the parasite to complete its liver stage development and make the cell type that can infect red blood cells.
By using fluorescent markers, the team found that parasites genetically modified to not carry FC were able to invade the liver in mice but none of them were then able to pass through and enter bloodstream. Instead the parasites without FC simply failed to grow in the liver and were killed off by the immune system.
Previously the importance of the FC gene in the liver stage had been difficult to definitively demonstrate, partly because the FC gene is also needed by the parasite to survive in the mosquito.
Ordinarily then, an FC-free parasite would never reach the liver because it would never survive in the mosquito. That meant researchers couldn’t be sure whether FC was important at the liver stage or not.
The trick was to take advantage of the way the parasites reproduce once in the mosquito’s gut. When an FC-free parasite mates with a wild, or unmodified parasite, it inherits enough FC to survive in the mosquito. But when it later divides to migrate into the mosquito’s salivary glands, where the FC gene is no longer needed, half of the parasites will be left FC-free.
Previously these FC-free parasites had been impossible to keep track of after all this mixing. But now these parasites will appear fluorescent because they will still carry the fluorescent marker they originally inherited from their genetically modified parent.
Testing the technique
Doctoral student Upeksha L. Rathnapala engineered and tested the technique. She was able to modify the parasite by replacing the FC gene with florescent proteins bright enough to be easily tracked through the liver.
The first step was to grow a population of FC-free parasites by taking malaria from the bloodstream of infected mice and then adding DNA that would delete the FC genes.
To do this, a population of parasites was passed through an electric current that temporarily disrupted the cell walls enough for about 5-10 percent of them to take up the new DNA.
Inside the DNA she had also embedded a genetic structure that made the parasites resistant to an old anti-malaria drug. Once the drug was added to the population, all the parasites without the new DNA were killed, leaving a completely FC-free and red-glowing population.
These FC-free parasites were then launched into the malaria life cycle using mice as the hosts.
Why GM mosquitoes might not work against malaria
Commonly a mosquito will inject about 50 malaria parasites into a host, and of these usually just a few reach the liver—the others get lost and killed by the immune system. But once they reach the liver they quickly adapt and grow exponentially.
“The liver is a very nutrient rich-place with big cells to invade, so the malaria parasites can go crazy. After just three days in a mouse liver one malaria parasite can grow into tens of thousands,” says Goodman.
From the liver, these thousands of parasites enter the blood stream and start causing the symptoms of malaria—fever, sweating, and nausea. But when Rathnapala looked for the glowing FC-free parasites in the blood stream, there were none. Somehow the lack of FC was fatal to malaria once it reaches the liver.
‘It does work’
Using the fluorescent protein to specifically identify those parasites without FC meant that for the first time their development in the liver stage could be tracked, telling researchers exactly when the parasites died.
Each parasite life cycle was a two-to-three-month process of tracking the parasite in its different forms, and analyzing what was happening in the liver as well as in the mosquito gut and salivary glands.
“After all that day-to-day effort in the lab, and then having to wait all that time to see whether it actually works or not, it just makes your day to finally see that it does work,” says Rathnapala.
She has also made the day of malaria researchers seeking new and easier ways to expose the parasite’s vulnerabilities.
Stopping parasite invasion could fight malaria
In 2015 there were 212 million cases of malaria and 429 000 deaths, mostly children in sub-Saharan Africa. While the disease is curable, and efforts to control the Anopheles mosquito that spreads it have reduced the incidence of infection by 21 percent since 2010, the parasite’s ability to develop drug resistance remains a major problem.
Despite encouraging progress, a licensed vaccine is yet to be developed. Techniques like this one that can better arm microbiologists in the race for a vaccine and new drugs can’t come fast enough.
A paper describing the technique appears in the journal PLOS Pathogens.
Source: University of Melbourne