High levels of salt make toils toxic and gravely limit plant growth on approximately one third of the world’s cropland. New research uncovers how some kinds of rice cope, while others don’t.
Some farmers call it a “white cancer” because white crusts of salt often mark the surface of afflicted soils. The losses in global crop productivity amount to some $12-27 billion each year.
But some plants have mastered the trick of growing on salt-afflicted (or saline) soils by tolerating levels of salt that would stunt the growth of most other plants. And new research is now unlocking how these plants do it and the important role silicon plays.
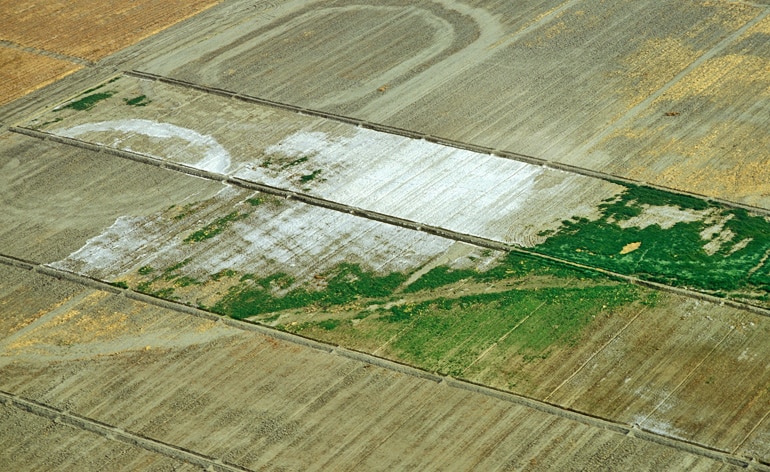
The work opens up the potential for new, high-yield crops that could thrive in salt-affected environments.
“Salinity has been a serious problem for agricultural production for many decades, and researchers have been desperately trying to work out how to help plants overcome the toxic effects of salt for nearly as long,” says professor Herbert Kronzucker, head of the School of Agriculture and Food in the University of Melbourne Faculty of Veterinary and Agricultural Sciences.
“Our team is working with physiological and molecular techniques to understand the cellular mechanism of salinity, and to ‘fish out’ the genes that are responsible for salt entry into plant cells. The ultimate goal is to learning how to control these in the world’s major crop species.”
What causes high salinity?
The cause of high salinity is usually through one of two routes, or a combination of the two.
“Primary salinity” results from the accumulation of salts over long periods of time, through natural processes like rock weathering or oceanic salt being deposited by wind and rain.
“One of our most important crops is rice, which feeds some three billion people worldwide.”
“Agricultural activities by humans have contributed in a major way to the salting of soils, in what we call ‘secondary salinity,'” says Kronzucker. “We have disturbed the water balance in the soil by clearing permanent native vegetation and replacing them with crops. The resulting imbalance in evapotranspiration often leads to excessive water removal, and the salts stay behind.
“In yet other areas, the addition of irrigation water in excess of what plants can use raises the natural water table, mobilizing salts previously stored in the subsoil up to the root zone. As well, often irrigation water is of poor quality and is contaminated by seawater.
“One of our most important crops is rice, which feeds some three billion people worldwide. So, in our laboratory, we have chosen rice as the main plant species to examine closely. Within the world’s many rices, there are some varieties that can tolerate elevated levels of salt while most others can’t, so it’s a really interesting case study.”
In the roots
For around 20 years, the scientific consensus has been that plants use protein-based ion pumps in root cells to move sodium away from sensitive parts of the cell. It is a process similar to how sump pumps move water out of your house when it is flooded.
Getting more food from poor soil “will be absolutely vital as the world’s population grows and soils continue to deteriorate.”
But Kronzucker and his team calculated that rice plants don’t possess sufficient internal energy reserves to run pumps like this with the intensity proposed in current cell-biology models, and so they started looking for other mechanisms.
They studied two types of rice, one salt-sensitive and one salt-tolerant, and grew them in solutions of nutrients that resemble saline and non-saline soils.
“We used radioactively labeled sodium (sodium-24) to follow the path of sodium, and found it entered the roots of both types of rice,” says Kronzucker. “But when we applied a range of chemicals that would halt any type of biological pump, the sodium still travelled through the plant, indicating the salt was moving by other routes.”
What the team concludes, as reported in the Journal of Experimental Botany, is that salt doesn’t actually move into the root cells much at all. Instead, the vast majority of it travels from the roots to the shoots in passageways between cells, in a sponge-like tissue called the “apoplast.”
“Salt-tolerant plants have much less sodium moving up into their shoots than salt-sensitive varieties, so there is something fundamentally different in the way sodium is transported,” says Kronzucker.
Protection from silicon
In a related experiment, the team also showed that the element silicon works to protect some plants from moving too much salt from the roots up into the sensitive leaves and flowers.
“When silicon was added to the plant’s growth solution, it decreased sodium movement to the leaves, most notably in the salt-sensitive rice,” says study coauthor Devrim Coskun from the University of Laval and the Canadian Centre for World Hunger Research at the University of Toronto.
“In the salt-tolerant cultivar (rice), silicon was also protective, but in a different way. It appeared to stimulate plant growth in general, which effectively diluted the concentration of sodium within plant tissues.”
What if plants could make their own fertilizer?
It appears that silicon may be involved in turning on genes that are responsible for the movement of sodium and/or water, and the team is now working on unraveling this process.
Silicon is the second most abundant element in the Earth’s crust, but it is usually found as silica (the main component of sand), which is a form plants aren’t able to utilize.
“A shortage of plant-available silicon is a significant issue in many agricultural soils, and it could be a part of the reason why salinity problems are so widespread,” says Kronzucker. “Modern precision agriculture techniques can be designed to allow for ‘nutrient poising’—that is a properly calibrated application of nutrient doses and ratios, precisely matched to plant demand.
“If we can understand more about how elements like silicon work to protect plants, we can boost it in soils that are low in plant-available silicon to protect against sodium toxicity.
“If we can also succeed in breeding in the genes that convey salt-tolerance, the technologies could work together to boost food production from poorer soils, which will be absolutely vital as the world’s population grows and soils continue to deteriorate.”
Source: University of Melbourne