A new method for loading iron inside nanoparticles creates MRI contrast agents that work better than the mainstay gadolinium chelates, which face increased scrutiny due to potential safety concerns, researchers say.
“The possibility of eliminating gadolinium exposure and getting a two-fold improvement in T1 MRI contrast performance is going to intrigue radiologists,” says lead researcher Naomi Halas, professor of electrical and computer engineering and of chemistry, bioengineering, physics and astronomy, and materials science and nanoengineering at Rice University. “When they hear we’ve done this with iron I expect they will be very surprised.”
“…this study is a perfect illustration of how differently things can behave when you engineer at the nanoscale.”
Contrast agents are drugs that improve MRI images and make them easier for radiologists to interpret. Radiologists can “weight” the results of an MRI and make specific tissues appear either brighter or darker by varying the conditions of the test.
Radiologists use two weighting techniques—T1 and T2. While they frequently employ iron-based contrast agents for T2 scans, there are few clinically available alternatives to gadolinium for T1 tests.
“Iron chelates aren’t new,” Halas says. “It’s widely believed they are wholly impractical for T1 contrast, but this study is a perfect illustration of how differently things can behave when you engineer at the nanoscale.”
‘Theranostic’ particles
As reported in ACS Nano, researchers created a modified version of nanomatryoshkas, concentric layered nanoparticles that draw their name from Russian nesting dolls.
Nanomatryoshkas and nanoshells, another layered nanoparticle Halas invented at Rice more than 20 years ago, are about 20 times smaller than a red blood cell and made up of layers of conductive metal and non-conductive silica.
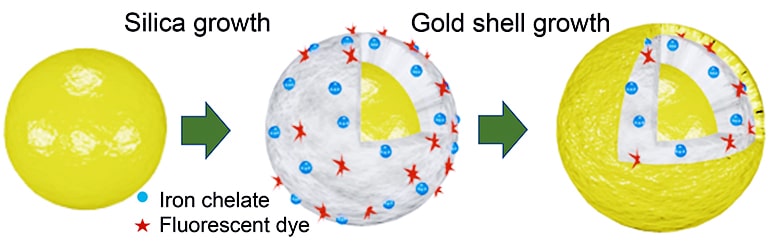
By varying the thickness of the layers, researchers tune the particles to interact with specific wavelengths of light. For instance, both nanoshells and nanomatryoshkas can convert otherwise harmless near-infrared light to heat. Researchers have used this localized, intense heating to destroy cancer in several trials of nanoshells, including an ongoing trial for the treatment of prostate cancer.
The new study is the latest chapter in efforts to create light-activated nanoparticles with a combination of therapeutic and diagnostic features. These “theranostic” particles could allow clinicians to diagnose and treat cancer in the same office or hospital visit.
More effective therapy
“If clinicians could visualize the particles through some sort of imaging, therapy could be faster and more effective,” says graduate student Luke Henderson, the paper’s lead author.
“For example, imagine a scenario where a scan is performed to verify the size and placement of the tumor, heat is then generated to treat the tumor, and another scan follows to verify that the entire tumor was destroyed.”
When Henderson joined Halas’ laboratory for nanophotonics in 2016, her team had already shown it could add fluorescent dyes to nanomatryoshkas to make them visible in diagnostic scans. Work was also underway on a study published in 2017 that showed gadolinium chelates could be embedded in the silica layer for MRI contrast.
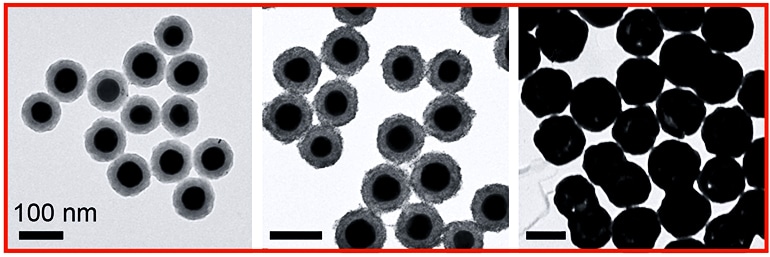
MRI scanners image the body’s interior by briefly aligning the nuclei of hydrogen atoms and measuring how long it takes the nuclei to “relax” to their resting state.
Relaxation properties vary by tissue, and by repeatedly aligning nuclei and measuring relaxation times, an MRI scanner builds a detailed image of the body’s organs, tissues, and structures. Contrast agents improve scan resolution by increasing the relaxation rate of particles.
Gadolinium chelates were introduced in the late 1980s and have been used more than 400 million times. Though gadolinium is a toxic metal, the chelating process covers each gadolinium ion with an organic wrap that reduces exposure and allows the drug to pass from the body via urination within a few hours.
Accumulation in brain
In 2013, Japanese scientists made the surprising discovery that gadolinium from contrast agents accumulated in the brains of some patients, and subsequent studies found similar deposits in bones and other organs.
While no adverse health effects have been associated with gadolinium-based MRI contrast agents, the FDA required drug makers to add warnings to the medication guides for eight widely used gadolinium-based contrast agents in December 2017.
“In the earlier work with gadolinium, we noticed that the nanomatryoshka design enhanced the relaxivities of the embedded gadolinium chelates,” Henderson says. “At the same time, we were hearing more calls from the medical community for alternatives to gadolinium, and we decided to try iron chelates and see if we got the same sort of enhancement.”
Not only was Henderson able to boost the relaxivities for iron, he was able to load about four times more iron into each nanomatryoshka. That allowed the iron-laden nanomatryoshkas to perform twice as well as clinically available gadolinium chelates.
“…the dyes were about 23 times more stable when they were inside the nanomatryoshkas.”
Henderson also found a generic way to change the type of metal that researchers could load. By adding unloaded chelate molecules to the silica first, he could load metal by soaking the particles in a bath of metal salts. By changing the metals in the bath, he could easily load different paramagnetic ions, including manganese, into the nanomatryoshkas.
After the metal ions were loaded into the silica, the researchers added the final layer of the nanomatryoshka, the outer gold shell. The shell, which is vital for plasmonics, also serves as a barrier to prevent ion leeching. The gold barrier also had a secondary benefit for the fluorescent dyes he added for dual-mode diagnostics, Henderson says.
“All fluorescent dyes are subject to photo bleaching, which means they fade over time and eventually won’t give off a measureable signal,” he explains. “Even if you freeze them, which slows down bleaching, they typically don’t last more than a couple of weeks.
“I was looking at an old sample of nanomatryoshkas that had been in the fridge for months, and I found they were still fluorescing quite well. When we looked more closely at this we found the dyes were about 23 times more stable when they were inside the nanomatryoshkas.”
Additional coauthors are from Rice, the University of Texas MD Anderson Cancer Center, and Mackenzie Presbyterian University in São Paulo.
The J. Evans Attwell-Welch Fellowship program from Rice’s Smalley-Curl Institute, the Robert A. Welch Foundation, the São Paulo Research Foundation, the National Cancer Institute, and the National Institutes of Health funded the work.
Source: Rice University