Scientists are a step closer to resolving a long-lasting head-scratcher: How do poisonous frogs keep from poisoning themselves?
To keep predators from eating them, some poison frogs, thimble-sized and dappled in cheerful colors, use the toxin epibatidine which binds to receptors in an animal’s nervous system and can cause hypertension, seizures, and even death.
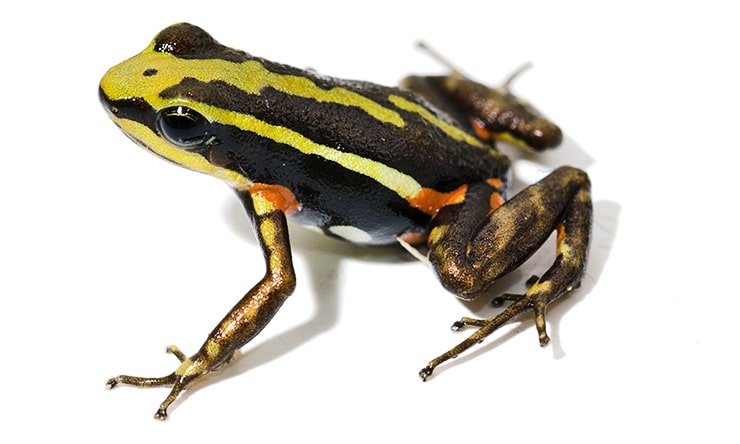
Figuring out how these frogs keep from being their own worst enemy could have potential consequences in the fight against pain and addiction in people.
Researchers discovered that a small genetic mutation in the frogs—a change in just three of the 2,500 amino acids that make up the receptor—prevents the toxin from acting on the frogs’ own receptors, making them resistant to its lethal effects.
“Being toxic can be good for your survival—it gives you an edge over predators.”
Not only that, but precisely the same change appeared independently three times in the evolution of these frogs.
“Being toxic can be good for your survival—it gives you an edge over predators,” says Rebecca Tarvin, a postdoctoral researcher at the University of Texas at Austin and a co-first author of a paper that appears in Science outlining the research.
“So why aren’t more animals toxic? Our work is showing that a big constraint is whether organisms can evolve resistance to their own toxins. We found evolution has hit upon this same exact change in three different groups of frogs, and that, to me, is quite beautiful,” Tarvin says.
There are hundreds of species of poisonous frogs, each of which uses dozens of different neurotoxins.
For decades, medical researchers have known that the toxin, epibatidine, can also act as a powerful nonaddictive painkiller. They’ve developed hundreds of compounds from the frogs’ toxin, including one that advanced in the drug-development process to human trials before being ruled out due to other side effects.
Skeleton key
The new research shows how certain poison frogs evolved to block the toxin while retaining use of receptors the brain needs and gives scientists information that could eventually help design drugs including pain relievers and those that fight nicotine addiction.
“Every bit of information we can gather on how these receptors are interacting with the drugs gets us a step closer to designing better drugs,” says Cecilia Borghese, another co-first author and a research associate at the Waggoner Center for Alcohol and Addiction Research.
A receptor is a type of protein on the outside of cells that transmits signals between the outside and the inside. Receptors are like locks that stay shut until they encounter the correct key. When a molecule with just the right shape comes along, the receptor gets activated and sends a signal.
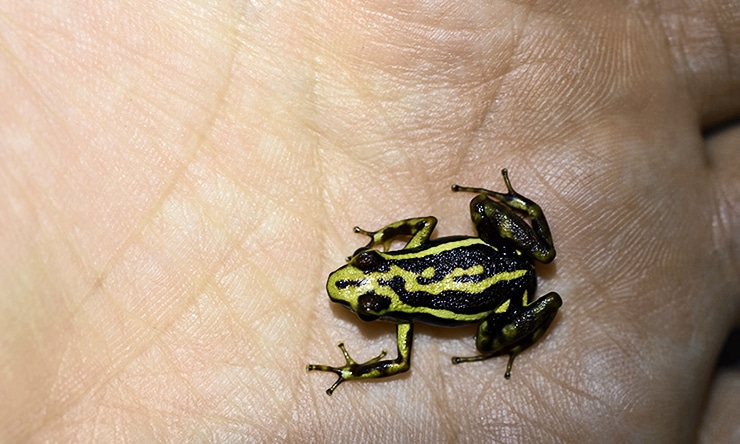
The receptor that Tarvin and her colleagues studied sends signals in processes like learning and memory, but usually only when a compound that is the healthy “key” comes into contact with it. Unfortunately for the frogs’ predators, toxic epibatidine also works, like a powerful skeleton key, on the receptor, hijacking cells and triggering a dangerous burst of activity.
The researchers found that poison frogs that use epibatidine have developed a small genetic mutation that prevents the toxin from binding to their receptors. In a sense, they’ve blocked the skeleton key. They also have managed, through evolution, to retain a way for the real key to continue to work, thanks to a second genetic mutation. In the frogs, the lock became more selective.
Pain and addiction
The way that the lock changed suggests possible new ways to develop drugs to fight human disease.
The researchers found that the changes that give the frogs resistance to the toxin without changing healthy functioning occur in parts of the receptor that are close to, but don’t even touch epibatidine. They studied the function of human and frog receptors in the lab of Adron Harris, another author of the paper and associate director of the Waggoner Center.
“The most exciting thing is how these amino acids that are not even in direct contact with the drug can modify the function of the receptor in such a precise way,” Borghese says. The healthy compound, she continues, “keeps working as usual, no problem at all, and now the receptor is resistant to epibatidine. That for me was fascinating.”
How ‘true frogs’ buck assumptions about evolution
Understanding how those very small changes affect the behavior of the receptor might be exploited by scientists trying to design drugs that act on it. Because the same receptor in humans is also involved in pain and nicotine addiction, the findings might offer ways to develop new medications to block pain or help smokers quit.
Working with partners in Ecuador, the researchers collected tissue samples from 28 species of frogs—including those that use epibatidine, those that use other toxins and those that are not toxic. The researchers then sequenced the gene that encodes the particular receptor in each species and compared subtle differences to build an evolutionary tree representing how the gene evolved.
Other researchers from St. John’s University and Stanford University are coauthors of the study. The National Geographic Society, the National Science Foundation, the UT Waggoner Center for Alcohol and Addiction Research, the National Institutes of Health, and seven academic societies funded the work.
Source: University of Texas at Austin